Optical Transceiver: Channel Configuration, Modulation Schemes, and Future Development
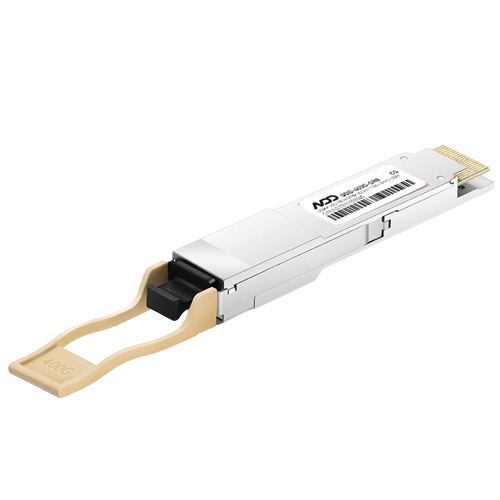
With the growing demand for data transmission, optical communication technology is gaining increasing attention as a high-speed, high-bandwidth solution. In optical communication systems, the design of optical transceivers is of significant importance as it involves the crucial task of converting electrical signals into optical signals for transmission. In the design of optical transceivers, the selection of channel configuration and modulation schemes is a critical decision factor.
This article explores the channel configuration, modulation schemes, and future development trends in optical transceiver design in three main sections, aiming to provide readers with a better understanding of the key points and cutting-edge technologies in optical transceiver design:
1. Design Routes: Single-channel, Multi-channel
2. Modulation Schemes: NRZ, PAM4, Overclocking, Coherent (QPSK/16QAM/64QAM, etc.)
3. Future Development: Ever-increasing data rates, Optical engines, Silicon photonics, etc.
1. Design Routes
The choice of design route depends on the packaging form and the type of laser selected based on the usage environment. Following that, the channel count and modulation scheme need to be determined based on the required transmission rate. In the design process, there are several critical indicators that cannot be simultaneously optimized, resulting in various design combinations.
These include chip-level performance and cost, channel count (miniaturization, heat dissipation, packaging complexity), and modulation scheme (chip cost, reliability, design complexity). The final design of the optical transceiver is determined by balancing and making trade-offs among these factors.
1.1 Single-channel
The simplest design approach involves incorporating a single laser and a single receiver within an optical transceiver. This configuration, along with other optical components and various electrical chips on the PCB, forms a single-channel optical transceiver.
For instance, a common example is a 10G optical transceiver using NRZ modulation with a single 10G chip, a 25G forward transmission optical transceiver using overclocking modulation with a single 10G chip, or a 100G DR1 data center optical transceiver using PAM4 modulation with a single 50G chip. These are all examples of single-channel design routes.
1.2 Multi-channel
As the difficulty of upgrading laser chips is significant, the highest mature level currently achieved is a single-wavelength 50G data rate. However, customers' bandwidth demands are rapidly increasing, with 400G and even 800G on the agenda. To address this, a multi-channel design approach has been developed, utilizing multiple lasers and receivers combined to achieve higher transmission rates.
However, the multi-channel approach faces the challenge of how to connect with optical fibers for transmission.
1.2.1 Multi-fiber Approach
The simplest approach is to directly transmit each laser through a separate optical fiber. This approach offers the advantage of a simple internal structure, requiring fewer components and resulting in lower costs. However, when multiple fibers are needed for transmission, the cost increases with the distance. Therefore, the multi-fiber approach is mostly used in medium-to-short-distance scenarios. Examples include 400G QSFP-DD SR8/DR4/XDR4 provided by NADDOD.
For instance, the 400G SR8 optical transceiver uses a standard 16-core multimode MPO connector and consists primarily of transmit and receive optical components, VCSEL driver, trans-impedance amplifier (TIA), PAM4 DSP chip (oDSP), controller, and other parts. Each transceiver contains 8 channels of optical and electrical components, compactly designed within the QSFP-DD package.
In the 400G DR4/XDR4 optical transceivers, the DSP converts 8x50G PAM4 electrical signals into 4x100G PAM4 signals, which are then transmitted to the optical engine. The DSP also serves as a clock and data recovery (CDR) unit, and each channel operates at a wavelength of 1310nm, requiring a separate optical fiber for each channel, totaling 8 fibers.
400GE-SR8
400GE-DR4/DR4+
1.2.2 Single-fiber Approach
For longer transmission distances, reducing the number of optical fibers becomes necessary. In this case, a single-fiber approach can be provided as an option for customers. The single-fiber approach utilizes the principle of wavelength-division multiplexing (CWDM), where 4 different-wavelength lasers are combined using a multiplexer (MUX) and transmitted through a single optical fiber. At the receiver end, a demultiplexer (DeMUX) separates the signals into 4 different wavelengths for detection.
This approach requires LC duplex interfaces, reducing the number of required fibers to 2. NADDOD offers 400G LC interface transceivers in QSFP-DD packages, such as 400G QSFP-DD FR4/LR4/ER4, to meet this demand.
2. Modulation Scheme Selection
The selection of modulation schemes is closely related to the design routes discussed earlier. In this section, we will directly compare the main modulation schemes and the additional electrical chips required to implement them.
2.1 NRZ Modulation
Traditional optical transceiver modulation is primarily based on NRZ (Non-Return-to-Zero). In NRZ modulation, the high and low power levels of the laser correspond to binary 1 and 0 signals, respectively. The NRZ principle is easy to understand. For example, if we want to transmit a binary signal: 100111, the actual emitted optical power from the laser would be as shown in the figure below. The power above the upper dashed line is interpreted as 1, while the power below the lower dashed line is interpreted as 0. In an optical transceiver operating in NRZ mode, only basic driver chips, amplifiers (TIA, LA), clock recovery (CDR), and main control chips (MCU or ASIC) are required.
2.2 PAM4 Modulation
To achieve higher data rates, introducing PAM4 (Pulse Amplitude Modulation-4) modulation technology is necessary, as directly upgrading the optical chip is difficult and costly. PAM4 modulation divides the power levels of the optical signal into four thresholds: below the lowest threshold is interpreted as 00, between the lowest and middle thresholds is interpreted as 01, between the middle and high thresholds is interpreted as 10, and above the high threshold is interpreted as 11.
By designing denser power level decisions, PAM4 enables the transmission of twice the data within the same time frame. Using the previous example of the binary signal: 100111, the optical power emitted from the laser would change as shown in the figure below.
Here, it is important to emphasize two concepts that are often confused. When we talk about the speed of a laser chip, it generally refers to the baud rate, which is the number of complete pulses it can send in one second (for example, a 25G EML chip can send 25*10^9 pulses per second, or even more considering link overhead, which is disregarded here). However, through high-order modulation techniques, one pulse can represent multiple binary data bits. The actual amount of data transmitted is referred to as the bit rate (for example, a 25G EML chip, after PAM4 modulation, is made into a single-channel 50G optical transceiver. When we say "50G PAM4 optical transceiver," it means the bit rate is 50G and the baud rate is 25G).
Because PAM4 modulation requires precise power control and narrower threshold determination, it has stricter requirements on signal interference caused by fiber dispersion. Therefore, most PAM4 applications require the use of EML (Electro-absorption Modulated Laser) lasers. Additionally, in addition to the NRZ driver chip, a signal processing DSP (Digital Signal Processor) chip is added (to convert digital signals in pairs into analog signals).
2.3 Overclocking Scheme
To reduce costs, overclocking technology has been developed, which involves using chips designed for lower speeds to transmit higher-speed signals. For example, in 5G fronthaul, a 10G baud rate optical chip is "forced" to carry a 25G signal.
The laser diode does not operate at full capacity immediately upon applying voltage. There is a ramp-up process. When we say a 10G chip, it means it can emit a complete 10*10^9 pulses in one second. However, the idea behind overclocking is simple. Even with this 10G chip, I insist on feeding it a 25G signal, expecting it to emit 25*10^9 pulses in one second. When continuous "1" and "0" signals arrive, the laser power has not reached its peak during the ramp-up phase before it starts to decrease. As a result, the quality of the overclocked signal deteriorates, and the recognition threshold decreases.
Currently, there are two main ways to compose 25G optical transceivers: 25G native optical chips and overclocked 10G optical chips. Compared to overclocked 10G chips, 25G native optical chips have the advantages of better reliability and higher stability. However, their mass production requires high process requirements, and the supply channels are mainly in developed countries. NADDOD chooses to use 25G native optical chips, which may not show significant differences in initial use but demonstrate outstanding reliability in the long run.
2.4 Coherent Modulation
The previous three modulation schemes are all based on intensity modulation, utilizing either the power intensity of the optical signal or the amplitude of a sinusoidal wave (carrier) to represent (modulate) binary signals (baseband signals). However, sinusoidal waves also have a phase parameter, and coherent modulation utilizes the principle of coherence to utilize both the phase and amplitude parameters.
Coherent modulation has two advantages:
1) It can transmit more data within one signal period (similar to the effect of PAM4 modulation).
2) It can achieve strong anti-interference capabilities by utilizing trigonometric functions and difference-of-product formulas (which we learned in middle school). Therefore, coherent modulation has irreplaceable advantages in long-distance transmission.
Due to the globally standardized form factors and interfaces of optical transceivers, customers generally specify the desired form factor. In simple terms, SFP/SFP+/SFP28/SFP56/QSFP28/QSFP-DD are all compact form factors corresponding to different interface speeds, while CFP/CFP2 are larger form factors that can accommodate more components and provide better heat dissipation.
3. Future Development
Now, let's discuss NADDOD's development direction.
3.1 III-V Photonics
3.2 Silicon Photonics
3.3 Coherent Optical Transceivers
3.4 CPO (Co-Packaged Optics) Technology
4. Conclusion
The channel configuration and modulation scheme of optical transceiver design are crucial for achieving high-speed and high-bandwidth data transmission. Through flexible channel configuration, we can meet the requirements of single-channel and multi-channel applications, improving data transmission efficiency and capacity.
In terms of modulation schemes, NRZ, PAM4, and coherent modulation (such as QPSK, 16QAM, 64QAM, etc.) each have their own characteristics and are suitable for different application scenarios. In the future, optical communication technology will continue to evolve, and the demand for transmission rates will never be satisfied.
Advances in technologies such as optical engines and silicon photonics will bring broader development opportunities for optical transceiver design, promoting the performance and integration of optical communication systems. By continuously pursuing innovation and technological breakthroughs, we can build faster, more stable, and more reliable optical communication networks to meet the needs of future digitized society.