Silicon Photons in Pluggable Optics
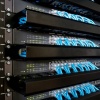
The never-ending demand for network bandwidth is the driving force behind the development of new technologies for data centers. Driven by the growth in the number of video applications, cloud services, gaming and connected devices, network operators are under pressure to upgrade and expand network capacity while minimizing the addition of new hardware. While the focus of hardware upgrades is primarily on network equipment such as routers, switches and servers, optical interconnections between these devices are becoming increasingly important in terms of both capital expenditures and operating costs.
The optical module at the end of the fiber optic link is the key driver of optical interconnect performance. Optical modules convert electrical signals to optical signals and back again. Optical modules are plugged into network devices and terminate fiber optic cables that run throughout the physical infrastructure of the network. Unlike ASICs and CPU chips, which act as the brains of the network and rely primarily on silicon-based transistors, optical modules rely on optical components such as laser diodes, photodiodes and optical waveguides to manipulate and modulate light to transmit information over fiber optic links.
This article focuses on the trend of building optical devices in silicon. So-called “silicon photonics” is expected to increase the integration of optical components and the spread of high-speed optics through high-volume production. Silicon photonics has long been of interest to the optical networking industry and has gained a foothold in data center networks in recent years. This technology is increasingly being used in high-speed networks as data center operators require larger quantities of optical modules.
Industry Background
Historically, the optics industry has been considered a niche market, especially when compared to the super scale of the silicon electronics industry. Over decades, the electronics industry has developed large-scale manufacturing processes based on photolithographically patterned silicon wafers. The large electronics market justifies economic investments in advanced fabs, material supply chains, automation tools, and design flows for developing and scaling electronic chips.
The optical communications market is much smaller than the electronics industry, and optical technology has evolved along a different technological path, using substrates such as gallium arsenide or indium phosphide rather than silicon wafers. Manufacturing technologies tend to be more customized in nature and do not benefit from the same economies of scale as electronics.
However, the mass production of optical devices has evolved significantly. During the telecommunications boom of the late 1990s, concerns about manufacturing scalability accelerated, and optical fiber was increasingly deployed in data centers because copper cables could not always support the required range at the highest data rates. Large-scale deployments of optics became particularly common in cloud and hyperscale data centers. At this point, high-volume manufacturing capabilities are critical to meet the demand for data center optics.
This is where silicon photonics becomes interesting, and after years of research and development, the technology has reached maturity. At the same time, the volumes driven by the optical module market are large enough to take advantage of the existing large-scale silicon manufacturing infrastructure. With the existing supply chain and commercial fabs, silicon photonics allows for the mass production of photonic wafers. Silicon photonics has matured to the point where it can be routinely deployed in real networks and provide value to end users. In the following, we will continue to describe the advantages offered by silicon photonics, citing examples of the technology base of silicon photonics.
Fundamentals of Silicon Photonics
Silicon photonics technology integrates the key photonic components and functions of high-speed optical modules into a silicon substrate that can be manufactured using standard commercial wafer fabrication facilities. Technology development in silicon photonics is focused on building and verifying optical components and designs that can be used in silicon fabs to produce photonic systems integrated in a single chip.
Optical modules convert electrical signals to optical signals on the transmitter side and convert optical signals to electrical signals on the receiver side. We can highlight the key optical components of the optical module by tracking the light coming in and out of the device. To receive the light, there must be a coupling interface to the silicon, which can be either vertically across the top of the chip via a grating coupler, or through the side of the silicon via edge coupling. A waveguide guides the light through the chip, and a silicon-based photodetector detects the light and converts the signal to the electrical domain, which is interpreted by the electronic portion of the device.
At the transmitter side, the light generated by the laser is guided into the chip, which then needs to be modulated into the signal connector that carries the information. The light is eventually coupled out of the chip and into the fiber. From there it can enter the fiber optic cable outside the optical module through a standard interface.
The light source is not integrated directly into the optical chip, a grating coupler under the light source couples the light into the chip, and the entire optical chip also uses passive photonic components to direct and manipulate the light.
Historically, optical devices have been discrete and based on substrates other than silicon, such as indium phosphide or gallium arsenide. In fact, different suppliers of the same component may even use different processes. However, if each component can be built in the same silicon process, fully integrated optical chips can be designed and fabricated to take advantage of the maturity and scale of CMOS manufacturing processes. Optical chips can be closely paired and integrated with the electronic chips that process the electrical signals. The figure below shows an example of a 100Gbps optical module with the entire optical module functionality contained in a single chipset. The value of this becomes apparent when optical devices need to be produced at extremely high scale.
Silicon Light Advantages
Manufacturing Efficiency and Automation
Reducing manual or custom processes helps increase factory throughput. Silicon Photonics offers a highly automated manufacturing process for optical devices involving high throughput processes and capital equipment. As mentioned earlier, the photonic wafers themselves are processed in the same commercial semiconductor fabs as electronic wafers. The integration of optical devices into a complete module can also take advantage of standard processes in the electronics industry.
The major assembly and test steps can be performed at the wafer level, so the process can be highly automated and output many units per hour. By leveraging wafer-level capabilities, it is easier than ever to expand capacity and produce millions of optical modules using this production process. And mass production of leading-edge optical modules (with data rates from 100G to 400G and beyond) can be adopted at scale within data centers.
As optical devices in general, and silicon optics more specifically, are adopted at shorter and shorter scales, volume will grow exponentially and gain more as overall deployment volumes translate into greater economies of scale. This virtuous cycle, along with the democratization of high-speed data center optics, makes silicon photonics a particular area of interest.
Wafer-level Testing and Module Yields
Silicon photonics brings additional benefits at a different level: reliability and repeatability. The design process for optical devices follows a workflow very similar to that of a traditional “fabless” electronics company. By defining the design of the optical device and compiling it into an optical device library and design suite, the final design can be fully defined and then etched into silicon by the fab. This results in much less variability in performance than traditional optical devices that may be affected by subsequent module assembly steps.
The maturity of photolithography and wafer etching used in silicon fabs allows for well-defined accuracy and repeatability of components. This means that statistical modeling and simulation can help to pre-understand and fully determine the performance of the optical module during the design process before the material is built. Of course, physical components can be built and measured to confirm the accuracy of simulation results. In this way, design-related problems can often be detected and corrected early in the product lifecycle.
Once the design is complete, silicon photonics has another unique advantage. Optical devices can be tested at the wafer level before they are cut into individual chips or assembled into modules. This brings several important benefits. If any components fail, they can be detected early in the build process; bad chips can be mapped and prevented from being built with other modules, thus avoiding wasting other components by using only known good chips. This has a positive impact on overall profitability, and this highly testable manufacturing process and repeatable design performance helps improve device reliability.
Economies of Scale
For silicon chip design, most of the hard work is put into the development and design phase of the product. Once money is invested in chip design and packaging, silicon can be repeatedly and efficiently stamped out. As the volume increases, the upfront fixed overhead of the design process can be divided into more and more units. As optical modules expand throughout the data center market, silicon photonic based optical devices will benefit from the rising volume curve and high speed optical devices will become more widely available in the market.
Integration with Electronics
While the primary application for silicon photonics is in pluggable optical modules, tighter integration with electrical chips and ASICs is actively developing, and future research is directed toward tighter integration of optical devices with switch ASICs, where current switch systems have long electrical traces from the switch chip to the front panel with pluggable optical ports. As signals get faster and faster, wiring from the switch to the front panel becomes difficult, and power consumption and heat dissipation are significant obstacles for system designers. If optical devices could be more tightly integrated with ASICs, the length of electrical traces between switch silicon and optical I/O would be minimized because signals would no longer be transmitted to the front panel.
In a world where cost-effective optical I/O, lower system power and higher bandwidth are required year after year, integrated optics reveals a path forward. The technical components and processes required to pursue an integrated optics approach will depend heavily on the maturity of silicon photonics. For direct-connected optical I/O, this is a promising long-term future. Even further, silicon photonics may eventually make high-bandwidth optical interfaces available for chip-to-chip interconnects, and some exciting technological developments are sure to continue to emerge in this area.
Summary
With the advantages of the silicon industry and wafer-level processes, silicon photonics defines the next generation of optical module manufacturing. It is proving critical to meeting the demands of the latest network architectures in the data center. As the maximum distance of copper links declines and is replaced by fiber, the demand for optical modules will continue to rise and the economies of scale will compound themselves.